Exploring the Evolution and Challenges of Elementary Particle Physics
Written on
Chapter 1: A Historical Perspective on Particle Discoveries
Commencing a lecture following his Nobel Prize win in Physics, Willis Lamb reflected on a notable quip: “While the discoverer of a new elementary particle was once celebrated with a Nobel Prize, the norm has shifted to imposing a $10,000 fine today—equivalent to $100,000.” This highlights the evolving landscape of particle physics and its recognition.
Nobel Prizes for New Discoveries
When the Nobel Prize was inaugurated in 1901, only two subatomic particles were identified: the electron and the proton. As researchers unveiled new particles, our comprehension of the universe beyond the atomic realm expanded significantly. Consequently, a growing roster of Nobel Prizes in Physics has been awarded for the identification and theoretical predictions of new "elementary" particles:
- 1935: Neutron
- 1936: Positron (the electron's antiparticle)
- 1949: Pion (the first meson discovered)
- 1959: Antiproton (the proton's antiparticle)
- 1976: J/psi meson (a novel type of meson)
- 1984: W and Z bosons (mediators of weak interaction)
- 1988: Muon neutrino (a new neutrino type)
- 1995: Tau lepton (a new lepton type, alongside electron and muon)
- 1995: Detection of neutrinos
- 2013: Higgs boson (responsible for mass acquisition in gauge bosons)
The initial detectors employed for particle identification were cloud chambers. These chambers utilized cosmic rays that collided with a supersaturated gaseous mixture, leaving identifiable trails of subatomic particles. From the 1920s until the 1950s, cloud chambers paved the way for the introduction of bubble chambers. Upgrading to denser liquefied gas, bubble chambers could detect higher-energy particles and were widely used until the 1980s.
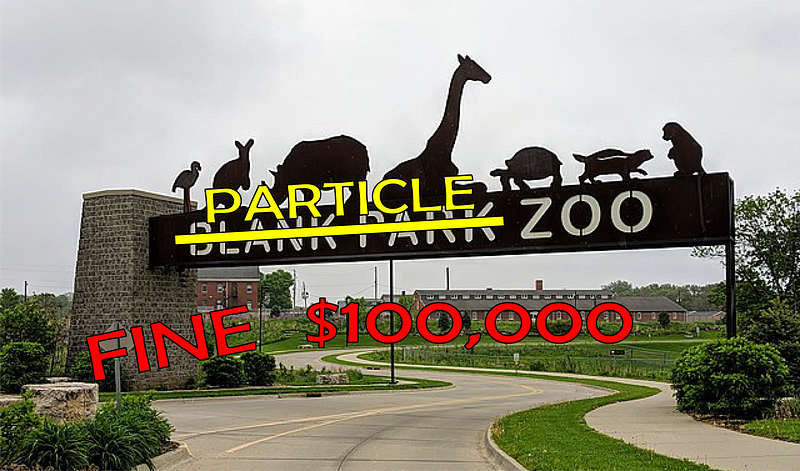
As time progressed, the realization dawned that the number of “elementary particles” exceeded earlier expectations. The advent of particle accelerators in the late 1930s and their operation in the 1950s further expanded our catalog of elementary particles.
The "Particle Zoo" Phenomenon
With an increasing number of identified particles, doubts arose regarding their true "elementary" nature. By the late 1960s, this overwhelming collection was informally termed the "particle zoo." At that time, quarks had yet to be discovered, leaving hundreds of hadrons classified as elementary particles.
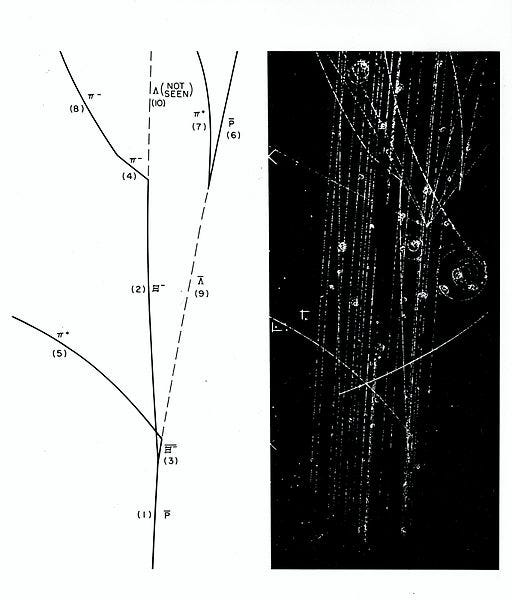
The resolution of the "particle zoo" dilemma came with the establishment of the Standard Model, which serves as a compact framework for understanding elementary particles. Nevertheless, the need for fine-tuning various parameters within this model (currently numbering 19) and the emergence of new unanswered questions evoke sentiments reminiscent of six decades ago.
Chapter 2: The Standard Model and Its Implications
The Standard Model is often likened to a periodic table for elementary particles. It delineates the components of matter and the forces that bind them. Grounded in symmetry principles, the model classifies particles into three categories: quarks, leptons, and bosons. Quarks and leptons form the matter, while their antiparticle counterparts (antiquarks and antileptons) constitute antimatter. The interactions occur through bosons, known as "force particles."
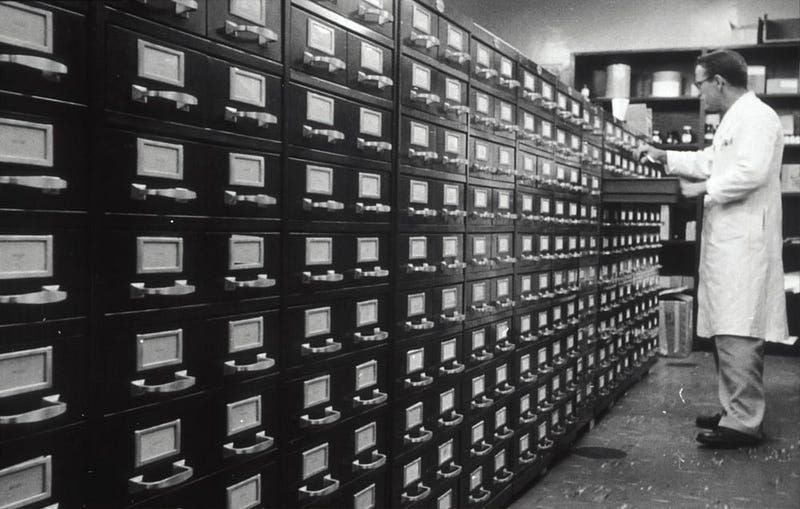
The creation of the Standard Model was not instantaneous; it evolved over many years, integrating new experimental data and pioneering ideas, many of which received Nobel recognition:
- 1957: Parity violation in weak interactions
- 1965: Quantum electrodynamics
- 1969: Classification of elementary particles and their interactions
- 1979: Unification of electromagnetic and weak forces
- 1980: CP-symmetry violation
- 1990: Development of the quark model
- 1999: Renormalizability of weak interaction
- 2004: Asymptotic freedom in strong interaction theory
- 2008: Links between CP-symmetry violations and quark generations
- 2008: Spontaneous symmetry breaking
- 2013: Higgs mechanism
Strengths and Limitations of the Standard Model
- Strengths: The Standard Model stands out for its mathematical consistency and precision, accurately predicting several particles later confirmed experimentally, including the top quark and Higgs boson. Its predictions align remarkably well with modern experiments.
- Weaknesses: However, the model falters at short distances and high energies. It successfully unifies three of the four fundamental forces but does not encompass gravity. While it incorporates special relativity, it lacks general relativity, rendering it an effective rather than a fundamental theory.
- Unresolved Questions: The Standard Model leaves several pressing questions unanswered:
- How does the model account for 95% of the universe's mass, attributed to dark matter and energy?
- Why is there a significant disparity between matter and antimatter in the universe?
- What underlies the mass of elementary particles, which the model fails to predict?
- What mysteries surround neutrinos, which appear to possess small, non-zero masses contrary to the model's predictions?
The quest for answers to these questions continues, shaping the future of particle physics and our understanding of the universe.
Further Reading
Explore additional topics covered in this series, including insights from a decade-long experiment on the muon magnetic moment, and ethical considerations in scientific research.
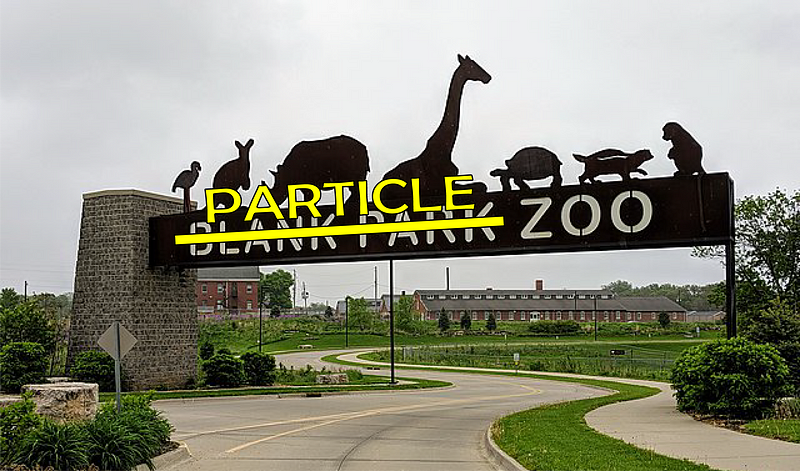